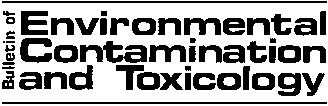
Bull. Environ. Contam. Toxicol. (1999) 62:34-39
© 1999 Springer-Verlag New York Inc.
Degradation of Sodium Monofluoroacetate (1080) and
Fluorocitrate in Water
L. H. Booth, S. C. Ogilvie, G. R. Wright, C. T. Eason
Landcare Research, Post Office Box 69, Lincoln, New Zealand
Received: 12 August 1998/Accepted: 10 November 1998
Sodium monofluoroacetate (1080) is a toxicant commonly used in New Zealand for the
control of vertebrate pests, principally the brushtail possum (
Trichosurus vulpecula) and
rabbits (
Oryctolagus cuniculus).
The widespread usage of 1080 and its high water
solubility have caused community concern about the environmental effects of the
compound and its potential for contamination of waterways. This has resulted in research
into the persistence of 1080 and its metabolites in water and the environment.
Previous research has shown that 1080 was degraded in stream water, but degradation can
be affected by many environmental factors. Parfitt et al. (1994) showed that 1080 was
degraded in biologically active water in 2-6 days while Eason et al. (1993) showed that
1080 declined by approximately 70% in 1 d and to below detectable limits (0.0003 ppm)
in 4 d in aquaria containing plants and invertebrates. Ogilvie et al. (1995, 1996) showed
that temperature significantly enhanced the rate of 1080 degradation, and that this was
further enhanced in the presence of aquatic plants and microorganisms. Some
microorganisms have been shown to degrade 1080 by cleavage of the C-F bond (Goldman
1965; Goldman et al. 1968; Walker and Bong 1981; Wong et al. 1992) using the adaptive
enzyme haloacetate halidohydrolase to produce glycollate (Fig. 1a). In animals, 1080 is
thought to be converted by the enzyme citrate synthetase to fluorocitrate (Fig. 1b), which
then inhibits the tricarboxylic acid (TCA) cycle via the enzyme aconitase.
Fluorocitrate
is the proposed toxic metabolite of 1080 in animals, but it is not known whether
microorganisms are capable of converting 1080 to fluorocitrate. Therefore, in order to
determine whether fluorocitrate is formed in the environment, we have developed a method
for the analysis of fluorocitrate in water.
MATERIALS AND METHODS
Stream water and the aquatic plant
Myriophyllum triphyllum were collected from the
Waimakariri River, Canterbury, New Zealand. Eighteen 2-L aquaria were maintained at
21 °C ± 2 with a 15/9-hr light/dark cycle. Aquaria contained either 1.8 L of stream water
and 60 g of plant material or 1.8 L of deionised water only (controls). All aquaria were
covered with polyurethane film to minimize cross contamination of the sterile controls with
microorganisms in aerosols, and were bubbled with air that had passed through a filter of
0.45 µm pore size.
Sodium monofluoroacetate was added to triplicate aquaria at 0.12, 2, and 5 mg/L, and the
Correspondence to: L. H. Booth
34
Figure 1b. Proposed biosynthetic pathway for formation of fluorocitrate by the TCA cycle
(Walker, 1994).
contents were thoroughly mixed on a magnetic stirrer immediately after the 1080 was
added, and again immediately before sampling. The lowest concentration of 1080 added
was 35 times higher than the highest concentration measured in field samples (0.0034 ppm)
collected after control operations (Booth et al., 1997), therefore the concentrations chosen
represent a worst-case scenario. Water samples of 100 mL were taken from all aquaria at
0, 12, and 24 hr, and 2, 4, 8, 14, and 17 d after the addition of 1080. All samples were
stored at -20°C and analysed for 1080 and fluorocitrate concentration.
Analysis was conducted with a 50 mL water sample which was acidified and the 1080 was
converted to the dichloroaniline derivative by N,N’-dicyclohexylcarbodiimide (DCC) and
2,4-dichloroaniline (DCA). The derivative was chromatographed on a silica cartridge,
eluted with toluene, and analysed by gas chromatography with electron capture detection,
based on the method of Ozawa and Tsukioka, (1987). This method has a limit of detection
of 1 x 10-4 mg/L in water.
Fluorocitrate analysis was conducted with a 50 mL water sample which was filtered
through a glass filter and evaporated to dryness. Residual fluorocitrate was derivatised with
boron trifluoride/trifluoroethanol at 75-80°C for 30 min. The sample was cooled, diluted
35
with water, extracted with toluene and analysed by gas chromatography using a Hewlett
Packard 5890 II gas chromatograph with a split/splitless injector and an electron capture
detector. The injector was used in split mode. The column was BP-5 column (30m x
0.33mm). The carrier gas was helium at a flow rate of 3 ml min -1. The injector temperature
was 200°C and the detector was 300°C. The column temperature was held at 60°C for one
minute, ramped up to 156°C at 4°C/min, then ramped up to 280°C at 25°C/min and held
for 10 minutes. Samples can be injected every 30 min. The retention time for fluorocitrate
is 15 minutes and the limit of detection is 0.01mg/L.
The rate of degradation of 1080 and formation of fluorocitrate was compared between
controls and sample aquaria using repeated-measures analysis of variance with
concentration as the categorical variable. The effect of initial 1080 concentration on 1080
degradation and fluorocitrate formation was also determined.
RESULTS AND DISCUSSION
Sodium monofluoroacetate was detected in all aquaria. The concentration of 1080 in the
aquaria containing stream water and
Myriophyllum triphyllum decreased over time to low
levels after 17 d (Fig.2). The rate of degradation was dependant on the initial concentration
of 1080 in the aquaria, with degradation occurring at a higher rate in aquaria with the
highest initial concentration of 1080 (p=0.021). In contrast, the control aquaria which
contained deionised water, showed no significant decrease in 1080 concentration over time.
These findings are consistent with previous reports indicating that microorganisms and
plants are responsible for the degradation of 1080 in aquatic environments (Ogilvie et al.
1995, 1996; Parfitt et al. 1994).
Fluorocitrate was detected in all aquaria containing stream water and
M. Triphyllum after
1 day (Fig. 3). No fluorocitrate was detected in the control aquaria. Fluorocitrate was only
detected in aquaria after 1080 degradation had started (Fig. 2), thus providing evidence that
fluorocitrate is a product of 1080 degradation. This indicates that microorganisms can
degrade 1080 in the same way as animals do, i.e., via the TCA cycle. Walker (1994)
suggested that microorganisms degrade 1080 using the enzyme haloacetate halidohydrolase
to produce glycollate and fluoride. Our data indicate that microorganisms are also capable
of converting 1080 in the environment to fluorocitrate. Fluorocitrate concentrations peaked
after 1, 4, and 8 d for the 0.12, 2, and 5 mg/L aquaria respectively and the amount of
fluorocitrate produced was dependent on the initial 1080 concentration in the aquaria
(p=0.001). Therefore, the fluorocitrate in the aquaria can only have been produced by
microbial degradation of the 1080 and the presence of plants and this supports the
hypothesis that microorganisms are responsible for conversion of 1080 into fluorocitrate.
Further evidence that fluorocitrate is a product of 1080 degradation is provided by the
relationship between the amount of 1080 degraded and the production of fluorocitrate over
time. Overall the amount of fluorocitrate produced was proportional to the amount of 1080
degraded (Table 1). At the time of peak fluorocitrate concentration 1 mole of 1080
produced 0.6-0.9 moles of fluorocitrate. After this peak, fluorocitrate was rapidly degraded
to below the limit of detection for the 0.12 mg/L and 2 mg/L aquaria and to near the limit
of detection for the 5 mg/L aquaria.
36
Figure 2. Degradation of 1080 in stream water aquaria over time. Error bars are ± 1
standard error.
Figure 3. Formation and persistence of fluorocitrate with time in stream water aquaria.
Error bars are ± 1 standard error.
37
Table 1. Mean amount of 1080 (µmol) degraded and fluorocitrate (FC) formed in
biologically active aquaria.
0.12 mg/L aquaria
2 mg/L aquaria
5 mg/L aquaria
(2.16 µmol 1080)
(36 µmol 1080)
(90 µmol 1080)
Time
1080
FC
1080
FC
1080
FC
(days)
0
0
0
0
0
0
0
1
0.8
0.7
19.0
4.1
1.0
2.7
4
1.2
0.2
19.0
11.0
9.9
8
1.4
0
32.7
9.3
21.0
15.0
17
1.6
0
38.6
0
52.0
0.1
This study has provided evidence that 1080 is degraded in stream water and that
fluorocitrate is a metabolite of 1080 degradation. Fluorocitrate like 1080 appears to be
rapidly degraded in water, so that within 17 d after dosing with 1080 there were either very
low levels, or no 1080 or fluorocitrate remaining in the aquaria. The implication of this is
that if 1080 is absent from a water sample collected after a 1080 control operation, then
fluorocitrate is likely to be absent also. Therefore it is legitimate to measure 1080 only, and
not fluorocitrate, when evaluating possible 1080 contamination of waterways after 1080
control operations. In addition, fluorocitrate has lower oral toxicity than 1080 (Peters et al,
1972; Savarie, 1984), due to its large molecular size. Fluorocitrate, therefore is not readily
absorbed and distributed throughout the body, in contrast to 1080 which is readily absorbed
and converted intracellularly to fluorocitrate (Peters et al, 1972; Savarie, 1984). In
conclusion, this research has shown that fluorocitrate is a product of 1080 degradation, but
it is rapidly degraded and due to its lower oral toxicity compared with 1080, it is more
important to monitor 1080 residues than fluorocitrate after a possum control operation using
1080 bait.
Acknowledgments. We thank The Foundation for Research Science and Technology for
funding this work, Richard Barker for statistical advice, and Eric Spurr, Dave Morgan,
Roger Parfitt, and Christine Bezar for editorial advice.
REFERENCES
Booth LH, Ogilvie SC, Wright GR, Eason CT (1997) Water quality monitoring after 1080
pest control operations. Water Wastes N.Z. 96:22.
Chenoweth MB (1949) Monofluoroacetic acid and related compounds. Pharmacol Rev
1:383-424.
Eason CT, Gooneratne R, Wright GR, Pierce R, Frampton CM (1993) The fate of sodium
monofluoroacetate (1080) in water, mammals, and invertebrates. Proc 46th NZ
Plant Protection Conf: 297- 301.
Eisler R (1995) Sodium monofluoroacetate (1080) hazards to fish, wildlife, and
invertebrates: A synoptic review. Contaminant Hazard Reviews Series, Report 30.
38
U.S. Department of the Interior, National Biological Service, Washington, D.C.
Goldman P (1965) The enzymatic cleavage of the carbon-fluorine bond in fluoroacetate.
J Biol Chem 240:3433-3438.
Goldman P, Milne GWA, Keister DB (1968) Carbon-halogen bond cleavage-III. Studies
on bacterial halidohydrolases. Biol Chem 243:428- 438.
McIlroy JC (1981) The sensitivity of Australian animals to 1080 poison. 1. Intraspecific
variation and factors affecting acute toxicity. Aust Wild1 Res 8:369-383.
Ogilvie SC, Bowen LH, Eason CT (1995) The effect of the plant
Myriophyllum triphyllum
and temperature on the degradation of sodium monofluoroacetate (1080) in an
aquatic ecosystem. Proc 48th NZ Plant Protection Conf:260-263.
Ogilvie SC, Hetzel F, Eason CT (1996) Effect of temperature on the biodegradation of
sodium monofluoroacetate (1080) in water and Elodea canadensis. Bull Environ
Contam Toxicol 56:942- 947.
O z a w a H , T s u k i o k a T ( 1 9 8 7 ) G a s c h r o m a t o g r a h i c d e t e r m i n a t i o n o f s o d i u m
monofluoroacetate in water by derivatisation with dicyclohexylcarbodiimide. Anal
Chem 59:2914-2917.
Parfitt RL, Eason CT, Morgan AJ, Wright GR, Burke CM (1994) The fate of sodium
monofluoroacetate (1080) in soil and water. In: Seawright, AA; Eason CT (eds)
Proceedings of the science workshop on 1080. The Royal Society of New Zealand,
Miscellaneous series 28:59-66.
Peters R, Shorthouse M, Ward PFV, McDowell EM (1972) Observations upon the
metabolism of fluorocitrate in rats. Proceedings of the Royal Society of London
183B:1-8.
Savarie PJ (1984) Toxic characterisation of fluorocitrate, the toxic metabolite of Compound
1080. Proc 11th Vertebrate Pest Conf:132-137.
Walker JRL (1994) Degradation of sodium monofluoroacetate by soil microorganisms. In:
Seawright AA; Eason CT (eds) Proceedings of the science workshop on 1080. The
Royal Society of New Zealand, Miscellaneous series 28:50-53.
Walker JRL, Bong CL (1981) Metabolism of fluoroacetate by a soil
Pseudomonas and
Fusarium solani. Soil Biol Biochem 13:231-235.
Wong DH, Kirkpatrick WE, King DR, Kinnear JE (1992) Defluorination of sodium
monofluoroacetate by microorganism isolated from Western Australian soils. Soil
Biol Biochem 24:8
39